Is There Really a STEM Workforce Shortage?
A robust science, technology, engineering, and mathematics (STEM) workforce is essential for the United States, serving as the foundation for effective employment, immigration, science and technology, education, and national security policies. Recognizing this importance, explicit government support for the development of scientific talent has long been a part of policy discussions. It was a pillar of Vannevar Bush’s 1945 report to President Roosevelt, Science, the Endless Frontier, which influenced the structure of America’s postwar scientific enterprise. And, since its establishment in 1950, one of the National Science Foundation’s eight enumerated functions has been to “maintain a register of scientific and technical personnel and in other ways provide a central clearinghouse for information covering all scientific and technical personnel in the United States.”
And yet, for almost as long, unsubstantiated claims that there is a significant shortage of STEM talent have been a running feature of STEM workforce policy discussions. In 1959, economists Kenneth J. Arrow and William M. Capron published an article responding to complaints of a shortage of scientists and engineers, noting that “in view of all the discussion of the ‘shortage’ problem, it is remarkable how little direct evidence is available.” Fifty-five years later, in 2014, demographer Michael S. Teitelbaum wrote: “The alarms about widespread shortages or shortfalls in the number of US scientists and engineers are quite inconsistent with nearly all available evidence.”
Frequently, the main stakeholder groups steering these conversations—businesses, universities, and government research agencies—benefit from the push to train and import more STEM workers. Others, including students and workers, rarely have their interests formally represented in these discussions. So even though numerous reports, analyses, books, and news articles have carefully examined demand and supply in the STEM workforce and labor markets over the decades and found no widespread or lasting shortages, perceptions of such shortages endure.
“The alarms about widespread shortages or shortfalls in the number of US scientists and engineers are quite inconsistent with nearly all available evidence.”
This persistent misunderstanding has limited policy choices and stymied serious policy discussions. Future employment projections are often wildly inaccurate and yet are regularly cited as precise predictors of the future. Unemployment rates are frequently mischaracterized and misinterpreted using the wrong benchmarks. And wage growth, the most direct measure of a mismatch between labor demand and supply, is often left out of the discussion entirely. Additionally, current high-level discussions lack nuance, failing to differentiate between the varied labor market dynamics across STEM fields, educational attainment, occupations, and geographies, preventing the development of well-targeted solutions. To move things forward, data must be used properly—with a recognition of their limits—and more careful analysis should be sought to better inform decisionmaking.
Employment projections
Workforce planning can be enhanced by estimating future needs, but the projections most often cited to support action for growing the supply of STEM workers—including by the National Science Board and even in the text of proposed legislation—come with serious caveats and limitations. The projected ten-year employment changes from the US Bureau of Labor Statistics (BLS) biennial Employment Projections (EP) report rely on a methodology that extrapolates recent empirical trends in occupations and industries to determine the projections. This method works reasonably well for occupations that have stable trends, such as legal occupations, and easily identifiable demographic determinants, such as home health aides. But BLS has no reliable way to identify factors that could alter future demand trajectories, such as technological innovation, offshoring, and abrupt shifts in consumption patterns.
For occupations in which uncertainty is significant, such limitations have led to an uneven track record. Figure 1 shows BLS projected job growth and actual job growth for computer and mathematical occupations over two time intervals. BLS overestimated job growth between 2000 to 2010 by more than a factor of three. In 2000, BLS was crafting its projections during the steep increase of the dot-com and telecom bubbles and did not—perhaps could not—predict the ensuing bust. In its 2010 to 2020 projections, by contrast, BLS underestimated growth by more than a factor of two. BLS crafted these projections during the unusually slow jobs recovery in the aftermath of the Great Recession, likely biasing the estimates toward the low end. The upshot is that BLS projections are highly unreliable for some occupations and should not, on their own, form a basis for assessing a forthcoming shortfall or making significant policy changes.
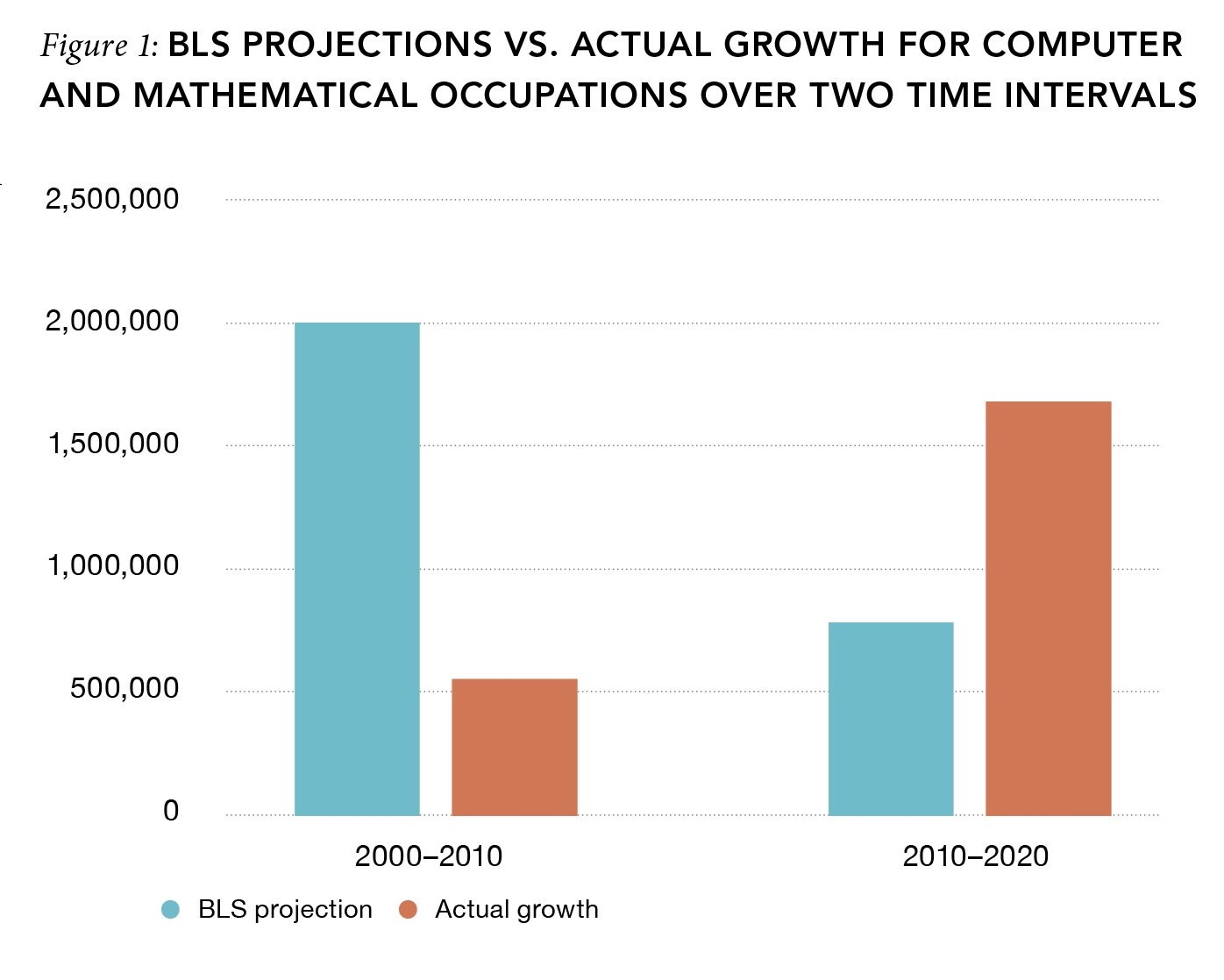
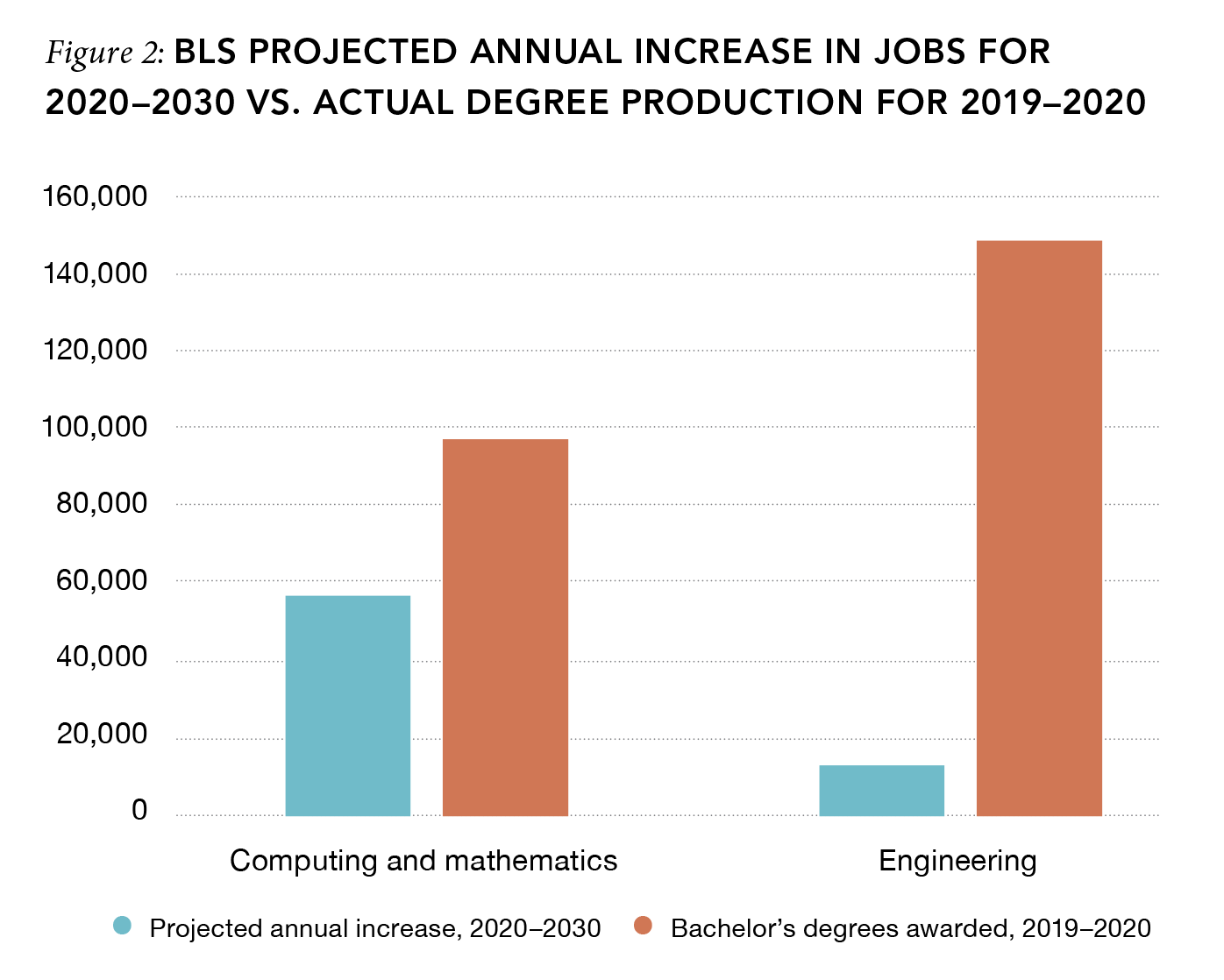
Moreover, these projected demand numbers are already unmatched with supply in terms of new degree production, as shown in Figure 2. Not every computer or math job requires a four-year degree. For example, computer support jobs, a sizable share of all computer jobs, typically do not require a bachelor’s degree. Comparing the most recent employment growth projections, from 2020 to 2030, with educational attainment in those fields right now indicates that roughly 569,000 of the 734,900 projected new jobs in computer and math industries will require a bachelor’s degree. This translates to an annual average growth of 56,900 positions, implying a demand for at least as many new workers in these occupations each year. But even in 2020, the number of bachelor’s degrees conferred in computer science alone was 97,047—40,147 more than the implied annual demand from the BLS projections. This mismatch is even more pronounced for engineering, where the number of degrees produced in a single academic year (148,120 in 2019–2020) exceeds not just the projected average annual growth, but the entire ten-year projected job growth from 2020 to 2030 (127,700).
On both the supply and demand sides, counting jobs is very difficult—and forecasting them into the future is challenging due to structural changes in the economy and other factors. Technological disruptions, and their effects on employment, are notoriously difficult to predict. For example, offshoring has drained millions of technology jobs from US demand. And industry growth is not the only factor that drives occupational demand; openings are also created when workers retire or switch careers, voluntarily or involuntarily. On the supply side, projections may be complicated by the fact that many computer and mathematical jobs are filled by workers with degrees in other fields. Finally, supply may come from outside the US pipeline, as in 2020 when employers imported 71,000 new foreign guest workers on H-1B visas for computer occupations, outpacing the predicted annual demand.
Technological disruptions, and their effects on employment, are notoriously difficult to predict.
A further misconception about employment projections is that they can be used to predict future labor shortages, even though BLS clearly warns to the contrary, saying that its “projections assume a labor market in equilibrium, i.e., one where overall labor supply meets labor demand.” Despite this caution, policy documents often use the projections to forecast such shortages, including legislation introduced by the ranking member of the House Science Committee in 2020.
Unemployment rates
Advocates frequently point to ostensibly low unemployment rates for STEM occupations as a strong indicator of shortages, but evidence shows those rates are actually high, not low. At a 2012 event on education and immigration reform, for example, Microsoft president Brad Smith, who was advocating for large increases in STEM worker supply, said, “If you look at the occupation that we know best, computer-related occupation[s], the unemployment rate is only 3.4%. Since the traditional definition of full employment is about 4%, that tells us that we have a shortage.” However, this reasoning was flawed because it used the national full unemployment rate as a benchmark for the rate for a specific occupation.
In fact, rather than indicating a shortage, a 3.4% unemployment rate is highly elevated for computer occupations, meaning these industries were in a jobs recession—not full employment—at the time. The national unemployment rate is a composite of all labor markets in the country, and it includes all workers regardless of geography, education, occupation, industry, age, race, ethnicity, and other factors. Applying the national benchmark of 4% broadly would indicate that all the occupations shown in Figure 3, including lawyers and accountants and auditors, are perennially experiencing severe worker shortages. For computer and mathematical occupations, according to an Economic Policy Institute policy memorandum responding to Microsoft’s claims, full employment yields an unemployment rate closer to 2%. Thus, at 3.4% unemployment, “there are too many educated, experienced STEM workers who are trying to find a job; there is not a shortage of them,” the memorandum states. A sustained unemployment rate far below 2% might point to a shortage of computer workers if it is accompanied by fast-rising wages. But neither condition has occurred in the past decade.
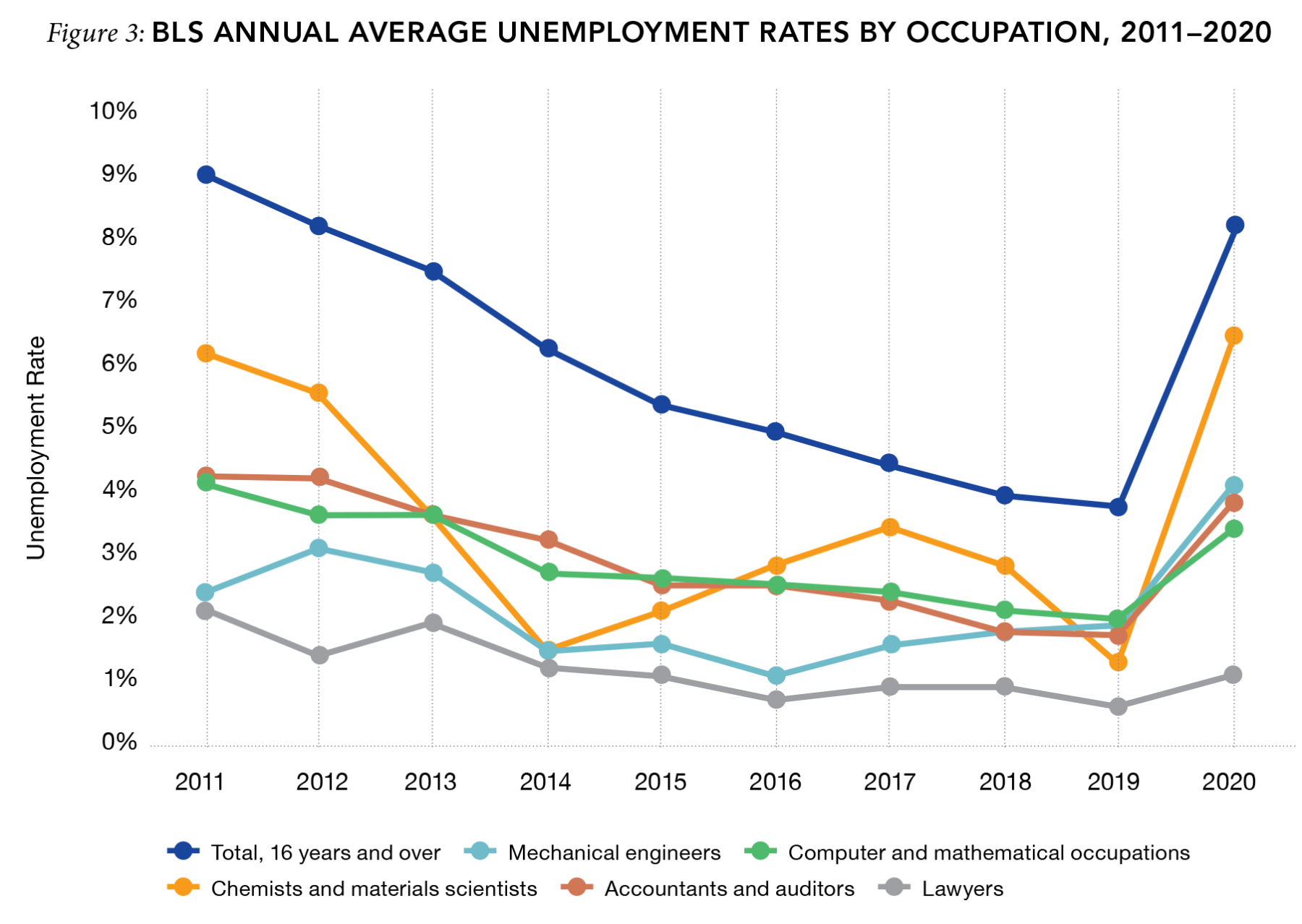
Wages and wage growth
The topic of compensation is noticeably absent from many discussions about the STEM workforce, even though it is arguably the most direct and important measure. In a market economy, as a 2001 National Academies of Sciences, Engineering, and Medicine study explains, “When demand exceeds supply in a particular occupation, compensation tends to rise relative to compensation in other occupations that require similar education, effort, and working conditions.” Simply put, when a shortage exists in an occupation, the relative earnings of those workers are expected to rise. Yet recent data show no such increases for many STEM jobs.
Although nominal wages increased for all workers between 2016 and 2021, the rates of increase for major categories of STEM occupations lag those for management and professional occupations and for all full-time workers, as shown in Figure 4. Moreover, after accounting for inflation, real wage growth was minimal or negative: real wages for computer and mathematical occupations declined by 0.4% over the five-year period.
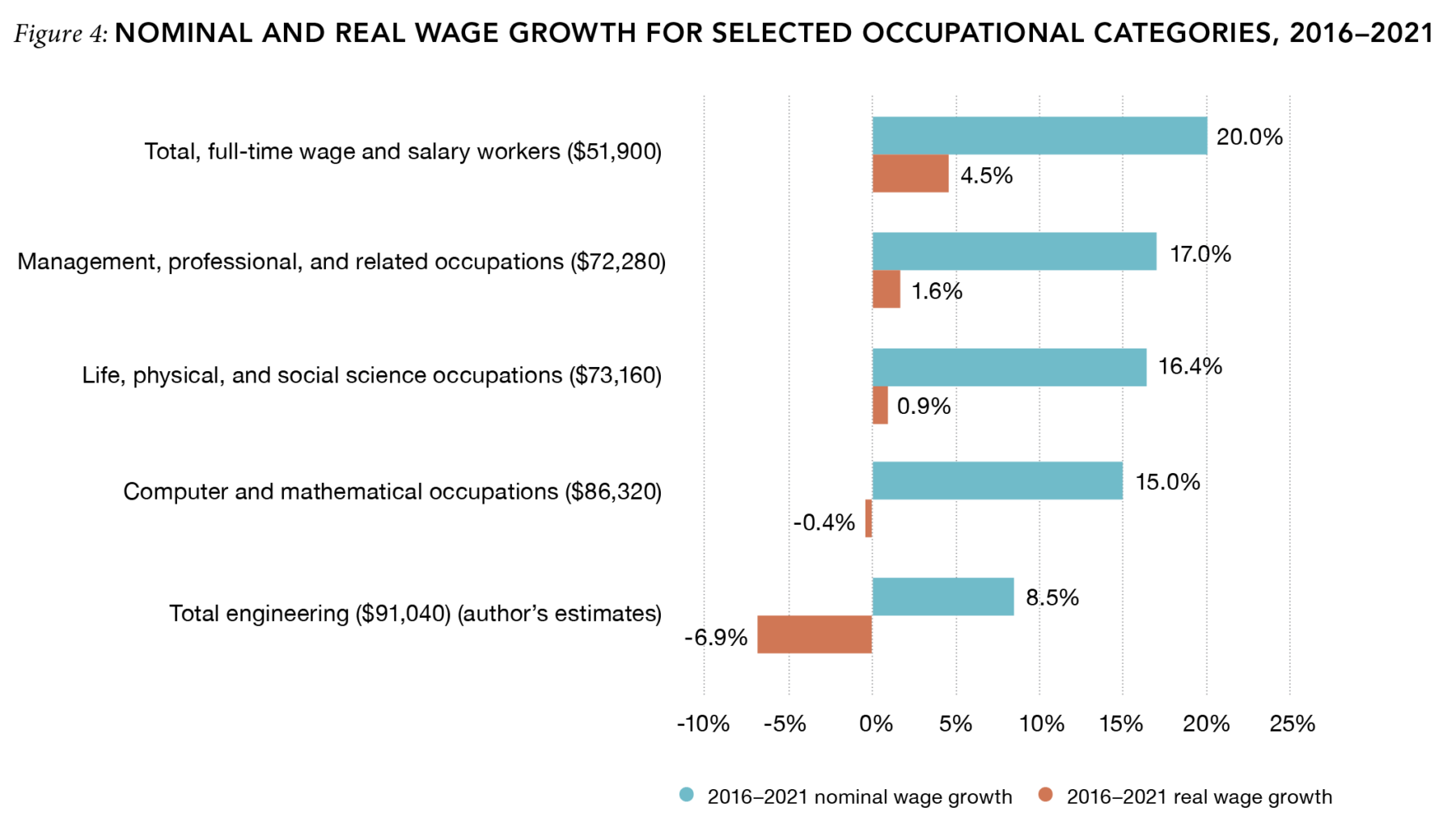
A more detailed look at specific STEM occupations in Figure 5 does not paint any rosier a picture. Only a few professions—medical scientists, chemists and material scientists, computer programmers, and physical scientists—saw both nominal and real wage growth exceed those of management and professional occupations and all full-time workers. Real wages declined for all types of engineers as well as for several other STEM occupations, including software developers, the largest and highest-skilled segment of computer occupations. While these remain high-wage occupations, those wages have stagnated or even declined. By contrast, accountants and auditors saw a modest increase in real wages of 1.2%, while lawyers gained more, with 3.3%—although both lagged wage gains for all workers.
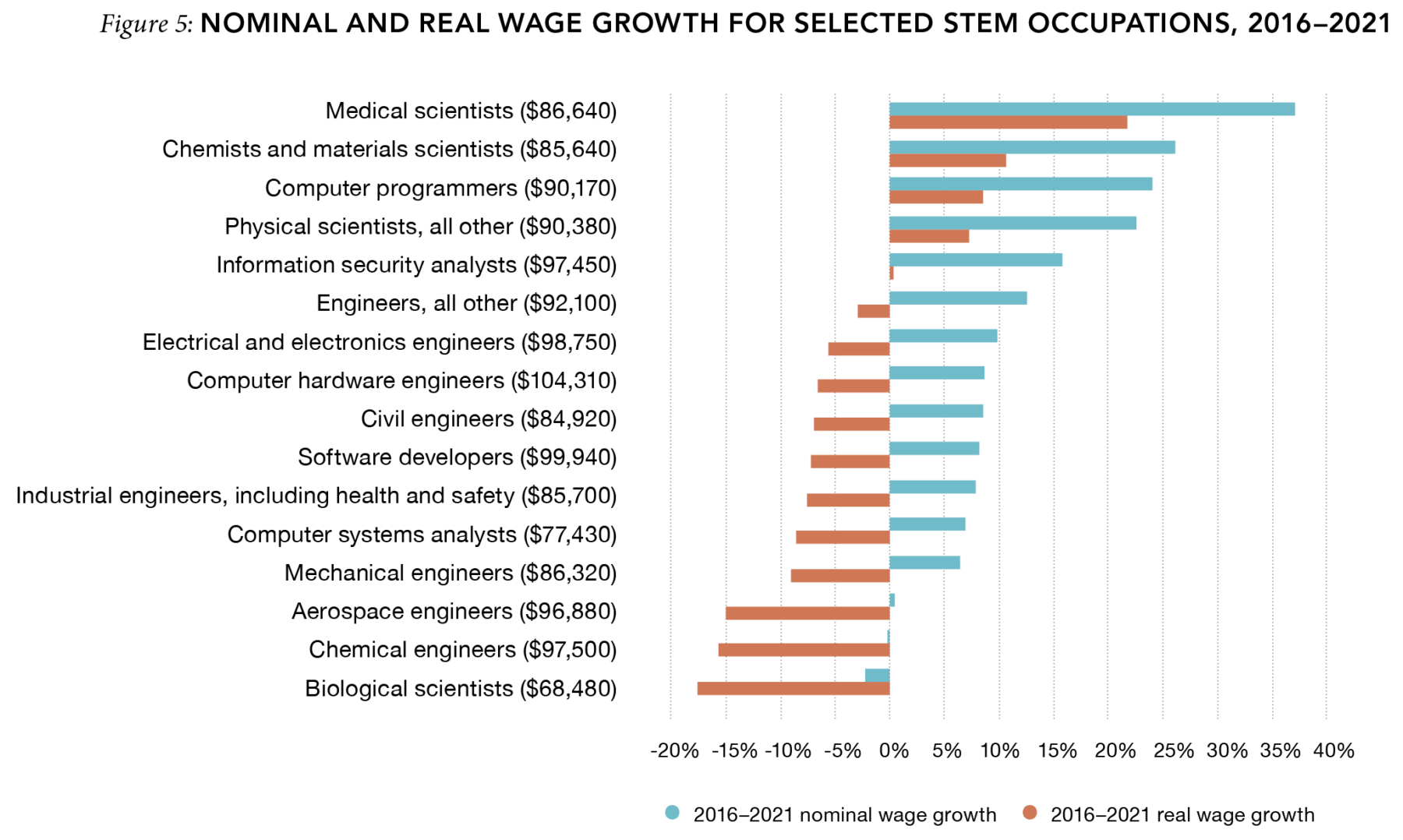
These trends are not new. The Congressional Research Service analyzed STEM wages from 2008–2012 and again for 2012–2016, finding that although STEM wages grew slightly during those intervals, wage growth deviated little from the stagnant rate for all occupations. These wage data make it challenging to argue there are serious STEM worker shortages, a conclusion reinforced by other price signals; for example, companies are able to fill an increasing share of their technology workforce as lower paid contractors. If technology employers were facing shortages, they would broaden their talent pool, achieve much better workforce diversity outcomes, and invest large sums into workforce development.
The imperative for a new conversation
The long-standing policy conversation over STEM workforce shortages, which is dominated by a few interested stakeholders and focused on limited data, needs to change. Much is at stake, even beyond US economic competitiveness and national security. In particular, the case for progress on diversity, equity, and inclusion—and for general improvements in the quality of life for American STEM workers—stands on its own.
However, a 60-year track record shows the situation will not change without concerted efforts by the larger science policy community. Rather than assuming looming shortages, the conversation would benefit from systematic investigation of whether shortages exist and in which fields, and how today’s complex labor market can be more effectively characterized. Only then can policymakers and others explore the best route to addressing these issues.
Rather than assuming looming shortages, the conversation would benefit from systematic investigation of whether shortages exist and in which fields, and how today’s complex labor market can be more effectively characterized.
Such investigation will require government agencies and foundations to invest in better data collection on and sound analysis of the STEM workforce. They also need to invest in infrastructure to ensure the resulting evidence is broadly disseminated. This is a challenge since civil society organizations that once played this role, such as the Commission on Professionals in Science and Technology, disappeared as their funding dried up. The public data infrastructure will get a significant boost if recent legislative and regulatory efforts to increase corporate transparency on employment demographics and pay are successful.
Concrete steps can be taken to improve the data. Analysts agree that STEM labor markets are heterogeneous—the market for PhD life scientists differs from computer engineers, for example—and cyclic, with occupational demand experiencing stagnation and even contraction at times. There are opportunities to conduct more sophisticated analysis of human resources data collected regularly by firms to benchmark themselves against competitors, such as days positions go unfilled, offer-acceptance rates, and signing bonuses. The most important missing piece for understanding and identifying STEM shortages and their characteristics, experts believe, is more granular, standardized job vacancy data by occupation. Current data are not sufficiently detailed; the BLS Job Openings and Labor Turnover Survey, for instance, reports only at the major industry level, with no reporting by occupation. And finally, there is the question of whether current metrics still reflect the reality of jobs within the United States. Calls by the National Academy of Engineering and Congress for government agencies to identify which jobs were moving overseas in the 2000s were stymied by business interests, but policymakers should request this information. Systematic measurements of job offshoring are a critical piece of information that could reduce labor market uncertainties and enable better targeting of STEM investments.
Better data and more rigorous norms for talking about those data can move the conversation to a higher level. More nuanced discussions would benefit everyone: businesses and universities, students and workers, the policymakers trying to make sense of it all, and, ultimately, the US science and engineering enterprise.