Building a Bottom-Up Bioeconomy
Engineering biology could play a critical role in creating a sustainable, resilient, and equitable bioeconomy, but getting there requires reimagining industrialization itself.
It’s 2032 and biology, applied to some of humanity’s biggest problems, has transformed economies and societies around the world. In the decade since the COVID-19 pandemic, global supply chains for chemicals, materials, food, medicines, and energy have become shorter and more resilient—enabled in no small part by the growth of biomanufacturing through thousands of small biorefineries around the world. Applied engineering biology has solved many entrenched problems while also bringing fulfilling jobs home to local communities. Because goods and energy are increasingly derived from biobased and renewable sources, petroleum use is sharply declining. Renewable resources—crops and algae, as well as waste materials and recycled gases—are sustainably managed to preserve biodiversity and minimize carbon emissions and pollution. This bottom-up bioeconomy has also enabled distributed governance systems that empower communities to tailor new approaches to their particular situations, creating a break with the industrial patterns and practices of the past.
Reimagining the bioeconomy
Engineering biology—which combines biology, engineering, and information technology to produce biobased materials and products—promises to create sustainable biomanufacturing around the globe. Already, engineering biology has enabled the scale-up of virus testing and aided rapid vaccine development during the COVID-19 pandemic. Building on research in and tools developed by synthetic biology, engineering biology now aims to translate its approaches of biological design, building, and testing to a wide range of applications, processes, and products. The ambition is to not only transform products we already use, but also create new ones, making use of nature’s intrinsic diversity. Unlike today’s petroleum and petrochemical refineries, which are concentrated in a few locations, the technologies of a new bioeconomy could be developed and deployed in a targeted manner, making use of local renewable and sustainably sourced feedstocks.
The pandemic, and the problems it has exposed, provides an opportunity to transition to an industrial ecosystem that better meets society’s changing needs. New approaches enabled by advances in engineering biology could be used to bolster more equitable and resilient societies and foster sustainable “circular” economies that can reduce waste and pollution, reuse materials, and more readily address climate and other environmental challenges.
The technologies of a new bioeconomy could be developed and deployed in a targeted manner, making use of local renewable and sustainably sourced feedstocks.
Engineering biology can help to accomplish the technical side of this vision, but it requires concerted action by policymakers, researchers, businesses, and communities to achieve its societal and environmental potential. Enacting this vision for engineering biology will also require a change in the field itself, so that practitioners come to see their role as more than simply engineering microbes, and instead embrace a larger mission of integrating diverse disciplines and knowledge sources while nurturing local capacity. Without deep social engagement, the field risks repeating the mistakes of the past and reinforcing currently inadequate economic and ecological systems. Reimagined in this way, engineering biology could play a critical role in realizing not just a vibrant bioeconomy, but a sustainable, resilient, and equitable one as well.
The state of the field today
To date, engineering biology has generated scientific breakthroughs, deployed CRISPR gene editing technologies, and started to bring biomanufactured products to market. But it has yet to deliver on its broader promises of transformative change toward more environmentally sustainable economies and societies.
Some engineering biology products are making early inroads into specialty markets. These products are beginning to offer not only “plug-in” alternatives to current products but also new processes, materials, and features. For example, tapping into rising consumer demand for plant-based diets, multiple companies are offering alternatives to animal products that use ingredients derived from engineered microbes and plants. Other companies are converting waste industrial gases and modifying proteins through biological processes into novel materials and textiles. Biological nitrogen fertilizers, which directly target genes in corn roots, have recently entered the market, replacing petrochemical fertilizers and avoiding nitrogen runoff. And in healthcare, cells from a patient’s own immune system have been engineered to attack cancerous tissues.
All of this is promising, and multiple initiatives are underway to accelerate biomanufacturing. An example is BioMADE, a US-sponsored biomanufacturing innovation institute created in 2021 with federal funding of $88 million. Internationally, the Global Biofoundries Alliance is a consortium of more than 30 public labs and facilities on four continents that seeks to speed engineering biology research and translation. Such public investments are providing a foundation for growing private sector investment. Private venture capital investment in synthetic and engineering biology has grown substantially in recent years, although much of the new investment has gone to health and medicine.
Without deep social engagement, the field of engineering biology risks repeating the mistakes of the past and reinforcing currently inadequate economic and ecological systems.
Yet, biomanufacturing still faces multiple challenges in generating transformative growth. These include scaling up the biological processes themselves, environmental concerns with feedstocks, entrenched competition from conventional sources, and regulatory instability. Importantly, biomanufacturers need to learn from past missteps: a decade ago, narrowly focused efforts to produce biofuels from sugarcane and other crops using genetically modified yeast ran into problems when they attempted to scale up. These efforts also raised significant concerns about feedstock supply and life-cycle sustainability.
As with any major technological innovation, a new biological industrial base will have to overcome technical production hurdles and demonstrate clear advantages over incumbent petroleum-based processes and products, including competitive pricing. What makes this more complicated than simply scaling production to achieve lower prices is that petrochemical-based incumbent products have been granted a formidable advantage in the marketplace. Not only are externalities such as carbon emissions, air pollution, and water pollution not accounted for by the nations that produce and consume the most, but fossil fuel subsidies actively create an uneven economic playing field.
Because of these barriers, most of today’s emerging biobased producers focus on niche, high-value specialty chemicals, such as molecules used for fragrances, pharmaceuticals, and industrial applications in which biological processes can avoid the costly toxic hazards associated with conventional production methods. Until biomanufacturers make inroads into high-volume sectors such as plastics and chemicals, these sectors will remain heavily reliant on petrochemical feedstocks and supply chains will continue to be unsustainable and highly sensitive to upsets. Meanwhile, policies such as carbon taxes at levels needed to promote deep decarbonization and reward sustainable supply chain transitions have failed to materialize.
Regulatory uncertainty is another issue facing new biomanufacturing approaches. Regulatory systems for biotechnology products are currently complex, fragmented, and difficult to navigate—particularly for new and innovative companies. And there is a related concern that the promise of harnessing biology for desirable ends is matched by its capacity to do harm, whether through intentional misuse, accidents, unintended side effects, or reckless behavior. Welcome developments can be seen in growing attention to responsible innovation in the synthetic and engineering biology communities as well as in the multidisciplinary iGEM (International Genetically Engineered Machine) global competition for students, entrepreneurs, and community labs. But there is not yet a coordinated framework for biotechnology regulation, despite efforts in the United States since the 1980s to create one. Both US and global regulatory systems need to enable more proactive and adaptive approaches for ensuring safety and security. A set of comprehensive guidelines, coupled with a new approach to partnering closely with communities to develop sustainable feedstocks and trust, could go a long way to enabling a transformative bioeconomy.
Reconsidering bioengineering’s challenges in a new light
Until now, the challenges facing bioengineering—scaling, sustainability, incumbency, and regulation—have been considered discrete issues, handled by different groups of experts. Questions of social and environmental impact and equity have often been treated separately, with less priority and at late stages. To make more continuous headway toward reaching a broader bioeconomy vision, we believe it is time for a fresh, integrated, and holistic approach.
Petrochemical-based incumbent products have been granted a formidable advantage in the marketplace.
In contrast to the traditional models of engineering and manufacturing, engineering biology harnesses intricate systems that have evolved—and are evolving—through natural selection. The field itself has developed new ways of thinking and working as it encompasses diverse disciplines such as micro- and molecular biology, chemistry, engineering, automation, data science, business, and humanities. Such interdisciplinarity can serve as a springboard for new and unique perspectives to not only create products that replace petroleum-based ones, but also innovative products that better address global, societal, and environmental challenges.
Why has scaling up and out of engineering biology to industrial contexts remained an obstacle to progress? Of course, jumping from academic research to industrial scale requires more investment in advanced process technologies and intermediaries that are able to bridge these gaps. However, a much bigger challenge underlies this bottleneck: policymakers must address the very nature of industrialization to ensure resilience, inclusivity, and environmental and social sustainability. Rather than trying to industrialize biology, the real task is biologizing industry. Only by tackling this larger challenge of reimagining industrialization can society realize the full potential of biological production.
One way to move toward realizing this potential involves rethinking the biofactory. In particular, rather than imitating the centralized systems of current industry, biomanufacturing should be fostered as a distributed system. In this model, the production of biological products—chemicals, fuels, materials, and medicines—would occur in green biorefineries located close to local sustainable sources of microbial feedstocks and raw materials as well as end users. Such distributed biomanufacturing could use locally unique bioengineering solutions to flexibly make a range of products for users. This model would create local jobs and expertise, nurture relationships between communities and producers, and improve resilience by reducing dependence on global supply chains.
By bringing together networks of local enterprises with public biofoundries for early-stage development, and by accompanying these networks with regionally focused initiatives such as education and training, this model could create a broad range of biobased end products and bring economic value to many localities. A distributed approach to biomanufacturing offers opportunities for rural regions and for reindustrialization and job creation in places such as those in the US Midwest or northern England that have been hit hard by the loss and relocation of their traditional manufacturing industries.
Yet to scale up sustainably, biomanufacturing must be designed to integrate with local systems without overexploitation. This could occur, for example, by making use of sustainable feedstocks, such as regionally available renewable dry plant matter, or by “mining” urban waste for bioengineerable resources. This requires developing biorefining at an appropriate scale for local situations and demand. It also requires engineered microbes that use the varied feedstock types available in different localities and at different times of the year. In other words, to make sustainability the heart of the bioeconomy, the practice of bioengineering must change from trying to engineer a single feedstock into a single mass product (for example, engineering switchgrass specifically for biofuels) to creating platforms that enable agile biomanufacturers to use multiple inputs and create multiple products both in parallel and in series.
Rather than trying to industrialize biology, the real task is biologizing industry.
Replacing petrochemical-based production and consumption systems with biobased alternatives will not inevitably or automatically lead to more sustainable, less polluting systems. New initiatives must avoid “problem-shifting,” whereby dealing with one sustainability issue causes or intensifies another. Instead, projects should be developed with an eye on circular biomanufacturing. In these systems, biomass is sustainably grown or reclaimed for use, with attention to recycling or ensuring safe biological decomposition. Moving to a local model of production will create opportunities for communities to reduce long-distance transportation of feedstocks and finished products, use local waste, and engage in new types of agriculture. Biomass and food production must be considered in an integrated way to ensure they complement rather than compete with one another.
Ensuring a distributed and integrated approach to biomanufacturing at a global scale could reduce international inequalities, facilitate sustainable development, and contribute to net zero carbon emission goals. But, as with artificial intelligence and other disruptive technological developments, engineering biology could also exacerbate existing inequities. To be fully successful, the distributed design we recommend needs to be accompanied by a broadening of governance processes.
Assuring that this new bioeconomy is equitable will require extensive societal engagement at various levels. Of course, wherever biomanufacturing is planned, inclusive engagement by companies with communities will be essential. But engagement by bioengineers with policymakers, communities, and other stakeholders at national and international scales will also be important to work toward consensus about responsible innovation and appropriate regulation, and to assure consistency in governance and regulatory frameworks. Unless all actors in the engineering biology ecosystem work now to ensure that both costs and benefits are distributed fairly, there is a danger that biomass production will become the latest industry in which the Global North benefits by extracting the resources of the Global South. Developed countries should build up their own renewable bioresources as much as possible and deepen global collaborations through open knowledge sharing, training, and developmental partnerships, so that developing countries can progressively build their local capabilities for sustainable biomanufacturing.
Four priorities for realizing a reimagined bioeconomy
Up to this point, policies supporting bioengineering have focused largely on advancing the science of synthetic and engineering biology. Much more needs to be done to design and implement policies to support and address the real challenges inherent in scaling engineering biology to construct sustainable, resilient, and expanded bioeconomies that address societal needs. We propose four principles to guide policy development, with key actions required from different actors across the engineering biology ecosystem to implement these principles (see table).
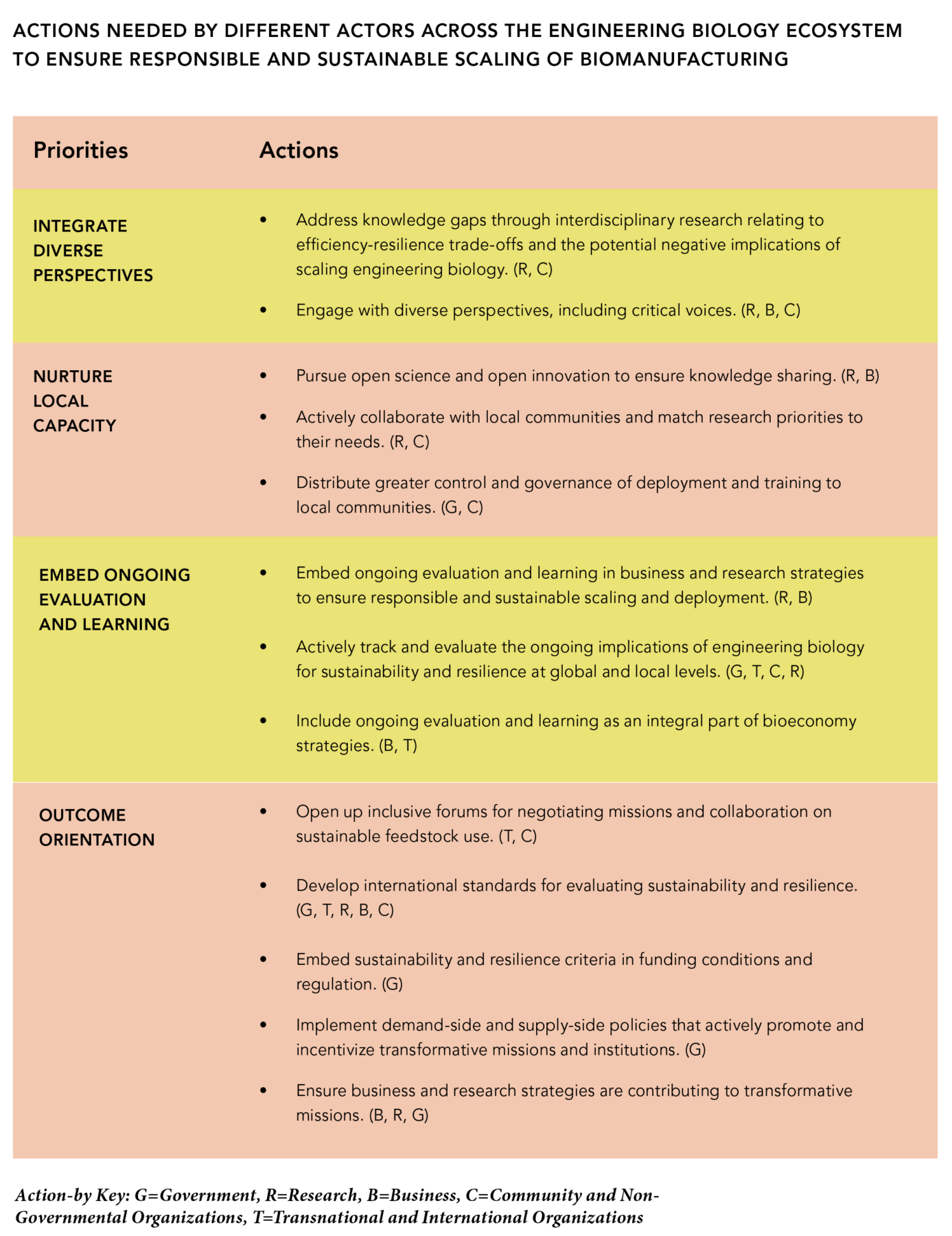
Integrate diverse perspectives. Disciplinary diversity is at the core of engineering biology, which combines biological understanding and design, data analytics, and engineering with environmental and social sciences. Yet, to avoid disruptive impacts on people, communities, and the environment, engineering biology must further broaden to encompass perspectives beyond the lab. This includes engaging with educators, communities, and citizens. As companies develop and deploy biomanufacturing applications, they must also embrace inclusive governance mechanisms to assure that the needs of people, as well as the planet, are embedded in the design and implementation of a reimagined bioeconomy.
Embed ongoing evaluation and learning. Scaling engineering biology will involve grappling with unpredictable technological developments, unexpected sustainability issues, and inevitable feedback effects as technologies are deployed within complex systems. To successfully address these challenges requires a cultural shift in the field toward experimental and open-ended approaches that embrace uncertainty and promote learning. Engineering biology will need to go beyond existing evaluation methodologies, such as life-cycle assessment, to create broader, more deliberative processes. Such processes must bring together stakeholders—including nongovernmental organizations, regulators, and communities—to explore uncertainties, reflect upon challenges, and decide new courses of action.
As with artificial intelligence and other disruptive technological developments, engineering biology could also exacerbate existing inequities.
Research funders and government agencies should ensure that evaluation and learning are integrated at multiple stages. During research, development, and commercialization, feedback can be used to determine which feedstocks are more environmentally sustainable. During and after the deployment of new technologies and systems, evaluation is critical to ensure that scaling is done in a responsible and locally sensitive way. Policymakers should make certain that bioeconomy strategies involve regular evaluation and reflection to ensure that progress is being made toward economic, social, and environmental goals. Funders, investors, and regulators have a critical role to play by explicitly setting criteria and standards for sustainability and resilience.
Nurture local capacity. For a distributed bioeconomy to provide high-quality jobs, it will be necessary to rethink how local labor is trained and valued. Federal, state, and local policymakers should work toward long-term integration of training and the development of career opportunities with the biomanufacturing industry. The industry also needs to articulate values beyond jobs, in framing how biomanufacturing supports societal well-being and communities.
We believe that two approaches will be helpful in achieving these goals. First, rather than a top-down scheme, new ventures should be community-led to ensure that locals are invested in the growth—including owning the biofoundries themselves. Host communities should also be involved in workforce training, community engagement, and the sharing of knowledge. This distributed system will require explicit consideration of biosafety and biosecurity. Although higher-level governance remains vital for national and international cooperation to tackle specific challenges around sustainability and security, a commitment to multilevel and local governance should be at the center of this effort.
Second, all stakeholders must commit to promoting open science and innovation. In engineering biology, access to data is vital, whether related to genetic information or reusable code for making biological components. Also essential is an intellectual property regime that does not overly restrict dissemination or raise barriers to entry yet protects traditional natural products from inequitable commercialization. The results of publicly funded research into core knowledge, tools, and data in this field should be open to all. Industry could be encouraged to share knowledge through consortia.
With ready access to research, data, and methods, a new generation of agile biorefineries can facilitate more localized and flexible knowledge exchange and production. Their spread could be further assisted through collaborations and partnerships across organizational and geographical boundaries to build a global network of local capabilities and infrastructures, renewable feedstock sources, and sustainable production and consumption models.
Be outcome-oriented. Rather than exclusively supporting bioproduction technology, policies should actively promote and incentivize the broader missions of building sustainable and resilient systems. In contrast to current strategies that focus on feeding the research and development, innovation, and venture capital pipelines, new initiatives must emphasize outcomes that are relevant to society, the environment, and the economy.
Part of building and scaling bioeconomies involves developing and implementing demand-side policies to encourage the purchase and use of sustainably manufactured biomaterials. Addressing the imbalances of present economic systems requires coordinated action across countries and sectors. Governments can incentivize demand by withdrawing subsidies for petrochemicals and stimulating new markets for biological products through price-support mechanisms and public procurement. For example, the United Kingdom’s use of the “contract for difference” funding mechanism, which protects renewable energy developers from electricity price volatility, is widely credited with the rapid growth of wind power generation, which is now achieving price parity with fossil fuels.
Policies should not promote bioproduction simply because it is “bio.” Rather, they should focus on bioproduction projects that are sustainable, resilient, and equitable.
However, such policies should not promote bioproduction simply because it is “bio.” Rather, they should focus on bioproduction projects that are sustainable, resilient, and equitable. To date, upstream R&D funding and government-driven supply-side approaches risk promoting technological alternatives that fail to achieve these desired missions. Explicit criteria regarding sustainability and resilience should be integrated into all policies and programs. Furthermore, members of business, academia, and government need to engage with each other and with social, environmental, and health organizations to address community needs for job security, wellbeing, inclusiveness, equity, and environmental sustainability. These important societal missions should be at the very core of business strategies, research agendas, and policy initiatives.
Delivering on biomanufacturing’s promise
Moving new biomanufacturing processes and products out of the lab and into the market is already challenging. Researchers and corporate leaders may worry that increasing the level of attention to broader societal and environmental challenges will make the job even more difficult. Yet such transformation is necessary—after all, most biomanufacturing starts with the promise of promoting sustainability and addressing global challenges but has often not delivered on that pledge. If biomanufacturing is to actively make a positive difference in addressing global challenges, benefitting society and the planet, it must explicitly make these ultimate aims part of the mission from the start.