Moving Beyond Hype on Hydrogen
Hydrogen has been “the fuel of the future” for a century. Whether it actually becomes an important part of a climate-friendly energy system depends as much on the cost of greenhouse gas emissions as it does on the tricky process of finding cheap, safe, and easy ways to harness hydrogen’s advantages.
Since 1923, when J. B. S. Haldane first suggested the use of renewable hydrogen in place of fossil fuels, hydrogen has been billed as a key player in the energy system of the future. But this future still hasn’t arrived, and interest in hydrogen has waxed and waned every few decades. In 2003, the George W. Bush administration announced a major hydrogen initiative through the US Department of Energy. But in 2016 Sir David MacKay, who served as chief scientific advisor to the United Kingdom’s Department of Energy and Climate Change from 2009 to 2014, wrote that “hydrogen is a hyped-up bandwagon.” MacKay noted that hydrogen production is very energy-intensive and the gas itself “gradually leaks out of any practical container.”
The 2021 Bipartisan Infrastructure Law directed $8 billion in funding to a program to build “hydrogen hubs” around the country. Is hydrogen’s long-forecast—and long-hyped—future finally here? And if it is, what makes this time different from those that have come before?
We think the answer to both questions lies in balancing hydrogen’s various potential advantages in a decarbonized energy system—as a fuel, as an energy carrier, as an energy storage medium, as a processes input, or as a combination of these—against its costs and risks. Those relative costs will be determined both by technological improvements and by whether policies are implemented that make emitting greenhouse gases to the atmosphere more expensive. Beyond these steps lie some normative questions, including whether decisionmakers support using fossil fuels to produce hydrogen after taking stock of economic costs and employment, social equity, and local environmental impacts. The development of seven hydrogen hubs throughout the country provides an important opportunity to test-drive not only whether hydrogen can be a good fit for future energy systems, but also whether society is comfortable with its potential complications and costs.
Looking over the hydrogen rainbow
Even though all hydrogen is the same gas—H2—it has been associated with different colors to describe how it was produced. “Grey” hydrogen is produced by splitting up a fossil hydrocarbon fuel and releasing the carbon dioxide (CO2) to the atmosphere. When carbon capture and sequestration is used to limit emissions to the atmosphere, the result is called “blue” hydrogen. The term “green” hydrogen is used when the molecule is produced with electrolysis powered by renewable sources of electricity to split hydrogen from oxygen in water. Some describe hydrogen produced with electrolysis as “pink” hydrogen if the electricity comes from nuclear power, “turquoise” hydrogen if it comes from a process that splits natural gas but produces solid carbon, “yellow” hydrogen if the energy comes from electricity obtained from the power grid, and so on. Deep geologic sources of hydrogen are often described as “white” or “gold” hydrogen, but today it is unclear whether such sources will prove to be sufficiently abundant or affordable to attract commercial interest. The US Department of Energy’s hydrogen hub program is now trying to move away from this rainbow of designations to simply call all the hydrogen the new hubs will produce “clean hydrogen.” Just how well this relabeling will sit with stakeholders is not yet apparent.
Is hydrogen’s long-forecast—and long-hyped—future finally here? And if it is, what makes this time different from those that have come before?
From a climate standpoint, what really matters for any given hydrogen production process is the amount of carbon dioxide—and other greenhouse gases—that is released to the atmosphere. Of the 10 million metric tons of hydrogen now produced in the United States, roughly 95% is grey, the product of steam methane reforming. This process produces carbon dioxide when natural gas, which is mainly methane (CH4), is split to produce hydrogen. If hydrogen produced in this way is to be used in large volumes in the future, it will become necessary to capture that carbon dioxide and dispose of it by injecting it safely and permanently into appropriate deep geologic formations, a procedure that is expensive, has yet to see widespread application, and may take longer to implement than many realize. While some observers argue that carbon capture and sequestration (CCS) is not a proven technology, it has been in use at commercial scale for almost 30 years at an offshore facility in Norway. Today there are roughly 40 CCS projects in operation globally and over 200 others in various stages of development.
Another question about production pathways is how much energy they require. With today’s technology, producing a kilogram of hydrogen from natural gas using steam methane reforming requires about 45 kWh/kg of energy. This level rises to about 50 kWh/kg with CCS, where the additional energy is required to compress the carbon dioxide and inject it deep into an appropriate geologic formation. The average energy to produce hydrogen using electrolysis is about 50 kWh/kg.
Assessing hydrogen’s possible advantages
Whether hydrogen ends up playing a central role in the decarbonization of the economy will depend on its cost and the speed with which, either directly or indirectly, users of fossil fuels must bear the costs associated with emitting greenhouse gases into the atmosphere. As the decarbonized grid and its financial structure evolve, hydrogen will only succeed if society is able to solve its problems in ways that are easier and cheaper than competing energy solutions.
Like electricity, hydrogen is an energy carrier, providing a way to move energy from where it is available to where it is needed. However, although electricity must be immediately used or stored in a battery, hydrogen can be more easily stored and used as needed, albeit at a cost.
Electricity has the advantage of decades of prior investment in high-voltage transmission lines, while hydrogen lacks dedicated transport infrastructure. Of course, electricity’s advantage will only continue so long as there is sufficient high-voltage transmission capacity. In its recent National Transmission Needs Study, the Department of Energy concluded that the United States will need to more than double regional and interregional electrical transmission capacity over the next several decades to meet decarbonization goals. However, it is difficult, sometimes even impossible, to build new conventional high-voltage transmission lines, with permitting and building often dragging on for a decade or more. Options for moving more electricity through existing transmission corridors are in nascent stages. Therefore, while existing transmission infrastructure is a distinct advantage for electricity today, ensuring the amount required in a decarbonized energy system will be a challenge of another order.
As the decarbonized grid and its financial structure evolve, hydrogen will only succeed if society is able to solve its problems in ways that are easier and cheaper than competing energy solutions.
In some situations, hydrogen’s ability to be moved in multiple ways—by pipeline or in pressurized containers by train, truck, or ship—may turn out to be an advantage over electricity. Unfortunately, natural gas pipelines cannot simply be repurposed because hydrogen can diffuse into steel pipes and cause embrittlement. It is possible to use other materials for pipelines to transport pure hydrogen; but even though siting pipelines can sometimes be easier than siting transmission lines, it is a slow and laborious process, and in the United States there is not yet a clear regulatory process. In some cases, moving hydrogen in gaseous or liquid form in large tanks on trucks, rail, barges, and ships could be a speedier alternative.
Another place where hydrogen might assist the evolving grid is in energy storage. Although battery technology is getting better, it is still expensive and in most cases not practical for storage durations of more than a few hours or at most a day or two. For longer-term storage, pumped storage hydropower or compressed gas storage can be an effective solution, but siting these large facilities is difficult. In contrast, hydrogen can be much more easily stored. Thus, for example, one potentially attractive application may be to make hydrogen from electricity when substantial wind or solar generation is available, store it, and then convert it back to electricity during periods when demand is high and wind and sun energy are not available.
This process, however, is not a simple solution to the problem of intermittent renewable generation because, to be cost-effective, the production, transport, and storage systems need to be used continuously, or nearly so. Thus, producing hydrogen only when there is excess wind or solar may not be economically attractive if expensive hardware must sit idle for extended periods. Likewise, producing hydrogen to immediately convert it back to electricity will be both expensive and inefficient. On balance, a range of emerging electrochemical storage solutions may prove cheaper and more efficient than hydrogen.
Barriers to “drop in” fossil fuel substitution
Hydrogen has sometimes been discussed as a “drop in” fuel to replace natural gas, but the reality is less straightforward. For example, although there are electricity-generating gas turbines today that can operate on pure hydrogen, measures must be taken to reduce nitrogen dioxide pollution because this pollutant forms at the high temperatures required for hydrogen combustion. While strategies exist to minimize resulting ambient air pollution, they add cost and complexity. Air pollution is not a problem if hydrogen is used in fuel cells because they rely on shuttling electrons rather than combustion to generate energy.
One potentially attractive application may be to make hydrogen from electricity when substantial wind or solar generation is available, store it, and then convert it back to electricity during periods when demand is high and wind and sun energy are not available.
Another hurdle for hydrogen as a replacement fuel is that it requires substantially more storage space for an equivalent amount of energy than, say, gasoline or jet fuel. In long-range aircraft, for example, where space is very limited, substituting hydrogen for conventional fossil fuels is very challenging. In some applications, this problem can be partly addressed by using hydrogen to produce a fuel, such as ammonia, that is more energy-dense. The shipping industry is seriously exploring using ammonia (NH3) for ship propulsion. Although ammonia is quite toxic, it could be used safely in commercial settings such as transport by ships or rail.
One place where hydrogen could replace natural gas would be as a source of high-temperature, tunable heat for industrial processes that are relatively difficult to electrify and thereby lower greenhouse gas emissions. Today, hydrogen is often considered superior to electricity for some industrial heat applications. However, as noted above, emissions of nitrogen oxides must be managed.
Hydrogen might be used to decarbonize some industrial processes, particularly iniron, steel, and chemical plants. In ironmaking, for example, coal, coke, and natural gas have traditionally been used to react with iron ore (iron plus oxygen), reducing it to pure iron (Fe). In the process, a carbon-based reductant, such as coke, reacts with oxygen to produce substantial quantities of CO2, which can be captured or directly emitted. When hydrogen is substituted for coal or natural gas as a reductant, for instance in the production of direct reduced iron, the process generates water vapor instead of CO2. This substitution shows promise as a way to dramatically reduce emissions from iron and steelmaking, although it is much more expensive than traditional methods because it requires large volumes of hydrogen as well as additional inputs of heat, for instance, to preheat the hydrogen.
In the absence of a price on greenhouse gas emissions, cost is the greatest barrier to using hydrogen in iron and steel production today. Carbon capture and sequestration may be a cheaper way to decarbonize ironmaking, but costs and timeframes associated with developing carbon capture with deep geologic sequestration are uncertain. Alternative ironmaking technologies such as electrowinning—which relies on electricity—are emerging and may someday compete with hydrogen-based processes.
Hydrogen hubs: An opportunity to learn while managing risks?
Since the 2009 Waxman-Markey bill (H.R. 2454), which would have created a nationwide emissions trading system, failed in the Senate, there has been no attempt to build a systematic national constraint on emissions of CO2 and other greenhouse gases. However, state efforts in California, Oregon, and Washington have placed limitations on the use of fossil fuels. And in parts of the Northeast, the Regional Greenhouse Gas Initiative caps power sector CO2 emissions, resulting in a price on CO2 emissions that rose to around $14 per ton in 2023.
Recognizing that today there may be no politically feasible way to implement national constraints on carbon emissions, the Biden administration has been trying to move the country toward decarbonization by subsidizing a variety of activities. To accelerate the adoption of hydrogen, as part of the 2021 Infrastructure Investment and Jobs Act (Public Law 117-58, also known as the Bipartisan Infrastructure Law), the Department of Energy received $7 billion to support between 6 and 10 regional clean hydrogen hubs, plus an additional $1 billion for cross-cutting activities. After a competition, DOE announced plans for seven hubs: in California, the Pacific Northwest, the Gulf Coast, Minnesota and the Dakotas (the Heartland Hub), the Midwest, the mid-Atlantic states, and Appalachia.
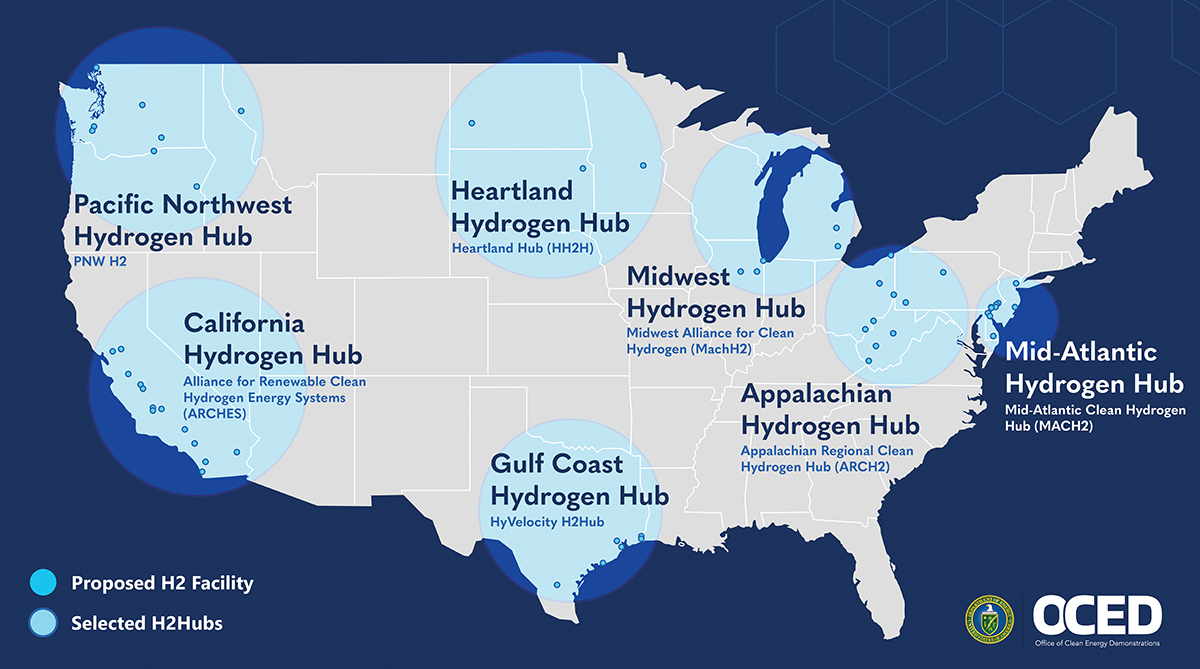
Source: US Department of Energy.
Most of these hubs will obtain some of the energy they need from renewable sources and many plan to produce hydrogen with electrolysis. The Appalachian Hub is expected to mainly produce blue hydrogen from natural gas. A portion of the energy for electrolysis for the Midwest and Gulf Coast Hubs will come from existing nuclear plants, however more hurdles will need to be overcome before the United States will be able to build significant additional nuclear power capacity.
In the absence of a price on greenhouse gas emissions, cost is the greatest barrier to using hydrogen in iron and steel production today.
The hydrogen these hubs produce will be used in a variety of ways. The Heartland Hub’s hydrogen will be used to produce fertilizer and to co-fire electricity generation plants. Some hydrogen from the Midwest hub will be used in the production of steel and glass. Several will support applications such as heavy transport.
The hydrogen hubs program has created a window of opportunity for the nation to test-drive hydrogen on a regional scale, giving communities and workers a chance to participate in developing the technology, along with supporting regulations and infrastructure. These hubs, positioned to foster regional collaboration, also have the potential to produce a diversity of experience that can inform a future effort to connect and scale hydrogen systems nationwide.
However, to reach their potential and identify workable arrangements for hydrogen in a decarbonized economy—and in the process win the support of an increasingly broad array of stakeholders—the hubs must do more than merely develop the technology; they must also de-risk it. In particular, they must focus on managing environmental impacts, creating markets and broad buy-in, serving and elevating the interests of communities and workers, and building public trust and acceptance through demonstrations of system safety. In many respects, the work of making hydrogen into a good neighbor may be as formidable as tackling the remaining technical and cost challenges.
Unless it is addressed with care from the very beginning, wider use of hydrogen could result in leaks of both methane and hydrogen—worsening climate change and ambient ground-level ozone pollution. Methane is roughly 30 times more potent than carbon dioxide as a greenhouse gas, though its lifetime in the atmosphere is shorter. In contrast, hydrogen is not a direct greenhouse gas, but once it enters the atmosphere, it extends the lifetime of methane. What’s more, hydrogen leaks more readily than other gases. For this reason, as hydrogen infrastructure is built out, doing so must avoid the kind of cavalier approach taken in building out natural gas, which has resulted in widespread leaks. Evaluating regulatory models and implementation in a hub context could provide templates for efficient, practical measures to be taken when hydrogen is used more widely.
The hydrogen hubs program has created a window of opportunity for the nation to test-drive hydrogen on a regional scale, giving communities and workers a chance to participate in developing the technology, along with supporting regulations and infrastructure.
The hydrogen hubs are being developed with theobjective of co-locating production and markets, to reduce dependence on single sources or uses. Policymakers have further emphasized the importance of placing hydrogen infrastructure in communities that face economic challenges, including places experiencing declines in fossil fuel production and related economic activity. The stated goal of these efforts is to ensure hydrogen hubs deliver broad benefits and, in the process, shore up political support for their continued existence and expansion. If they primarily benefit wealthy coastal economies, as in the case of electric vehicles, while economically challenged communities fall further behind, real and perceived inequities may increase local opposition to the low-carbon energy transition.
Making the energy transition inclusive requires that it create opportunities for workers and be responsive to the concerns of communities. It is important to note that these considerations will not always align, but should nevertheless be raised and considered in open and transparent processes. For instance, hydrogen production from natural gas would not only potentially allow a lucrative (for some) business to continue, but it would potentially create new jobs constructing and operating pipelines and sequestration sites that utilize many of the same skill sets of fossil energy workers, potentially easing workforce pressure in a transition. At the same time, communities may have mixed views of hydrogen, or—as research at Carnegie Mellon University suggests—may not have even heard of it, necessitating education and outreach early and continuously as the hubs are developed.
Another important concern in the development of hydrogen involves the safety of pipelines for hydrogen and captured CO2. If a hydrogen or natural gas pipeline leaks or breaks open, the escaping gas will rise and disperse fairly quickly. In contrast, CO2 is denser than air: in a low-lying location, the escaped gas can puddle, asphyxiating people and animals. This happened in Satartia, Mississippi, in February 2020, when a CO2 pipeline leaked, causing more than 200 people to be evacuated and at least 45 hospitalized, after emergency and other vehicles with internal combustion engines stopped functioning. Given that hydrogen is highly flammable, regulations for safe handling—especially for high concentrations in confined spaces—will be important. Even if hydrogen hub investments show early signs of financial viability and contribute to CO2 emissions reductions, a single pipeline disaster could cast doubt on the entire project.
A role for hydrogen in decarbonizing the US economy?
Whether hydrogen ends up playing a central role in the decarbonization of the economy will depend on the speed with which users of fossil fuels must bear the costs associated with emitting greenhouse gases into the atmosphere. It will also depend on hydrogen’s cost for each specific application and how its ease of use evolves relative to possible substitutes. And beyond those issues, it needs broad social acceptance and management of its risks and costs.
Making the energy transition inclusive requires that it create opportunities for workers and be responsive to the concerns of communities.
Clearly, there is a limit to how far the country can afford to go in subsidizing a decarbonized economy. However, creating hydrogen hubs is an important part of a strategy to ready the technology, explore its potential uses, and build workforces and regulatory frameworks to prepare for the future. Investment and production tax credits and funding for demonstration projects can work in concert with these efforts. Likewise timing—in development of the technology, reduction in costs, and fit into needs of the evolving grid—will be supremely important. Thus, in the absence of new policy that constrains greenhouse gas emissions, expiration of the hydrogen tax credits at the end of 2032 has the potential to undermine the economics of many hub activities.
If and when the United States succeeds in becoming more serious about decarbonizing, hydrogen alone will not be a silver bullet. At best, it will become an important part of the portfolio of technologies and strategies in an economy built on an increasingly clean electricity grid. At worst, it could end up as a passing fad, again. If we had to place a bet today, we would split our chips 70:30.