An Ambidextrous Approach to Nuclear Energy Innovation
Tension between the promise of new nuclear technologies and uncertainty about their feasibility requires a diversified, balanced research portfolio that can be adjusted locally in concert with global progress.
After many years of stagnation, a new wave of nuclear energy innovation is underway. Announcements of scientific and technical advances from laboratories and fusion and fission companies have become a regular occurrence. Each announcement seems flashier and more hopeful for the future of the industry than the last. With all this new activity, parsing the differences between the technologies in play—and evaluating their potential to transform the energy landscape—is as necessary as it is complex.
In the absence of a clear frontrunner among the various technologies, we argue that the appropriate response is a portfolio of nuclear energy innovation projects, informed by periodic assessments of technology readiness, potential benefits, remaining uncertainties, and economic competitiveness. Comparing new fusion and fission technologies within this kind of framework can inform policymakers, researchers, and investors about trade-offs between technologies. Adopting such a framework could also help decisionmakers understand how stakeholders might align in the pursuit of new forms of nuclear energy that can be deployed at scale.
The spectrum of nuclear energy innovation
Across the range of nuclear energy technologies, there are trade-offs among options for economic, technical, and political feasibility. Currently, all forms of nuclear power involve radioactive materials or have remaining physics uncertainties or face economic challenges—but in varying degrees. Today’s flurry of nuclear energy innovation is aimed at changing that, promising to make nuclear power production cleaner and cheaper. However, each of these aspirations comes at the price of different levels of uncertainty. This tension spans both fusion and fission research efforts.
Parsing the differences between the technologies in play—and evaluating their potential to transform the energy landscape—is as necessary as it is complex.
Depending on which dimension they value most, entrepreneurs, scientists, and investors arrive at different assessments of which approach yields an optimal ratio of potential benefits and remaining uncertainties. In our experience, advocates for each technical approach typically claim that theirs alone occupies a sweet spot, arguing that other projects are either too conservative and lack ambition or are speculative and unrealistic.
It’s also important to consider the ways that nuclear energy innovation agendas vary depending on social, cultural, and historical factors. France, for example, has a markedly different perception of, and tolerance for, nuclear fission than does Germany. Two-thirds of France’s electricity generation comes from nuclear reactors, and public support for fission technology is strong.
Germany, on the other hand, recently closed its last nuclear power plant and is unlikely to reverse course—largely due to a complex political history and public concerns about the radioactive materials needed and produced in the reactors. Consequently, Germany’s nuclear energy innovation agenda focuses on technologies with considerably lower associated radioactivity than traditional fission reactors.
For a comparative analysis of the variety of nuclear innovation efforts underway and to allow for better consideration of the trade-offs involved, we propose situating the various approaches in a design space with three axes: first, the amount of radioactive inventory and waste involved; second, the remaining scientific and technology uncertainty; and third, the expected economic competitiveness. Each fission and fusion approach can be located in this design space. A fine-grained analysis would treat radioactive inventory, radioactive waste, and release potential separately. However, in practice, the three are largely correlated.
The figure below is an example from our perspective of what this prospective analysis could look like. As we will explain in greater detail, more extensive analyses of this kind could be undertaken and may arrive at quantitative values for variables across the spectrum. Results of such analyses could then be debated in public forums and refined.
The figure makes it possible to see that the approaches with the largest amount of radioactive inventory and waste, such as traditional nuclear fission plants, are the most technologically mature. In turn, alternative approaches, such as fusion, that promise lower radioactive inventories and less waste have greater associated physics uncertainties. This schematic defines what we call the spectrum of nuclear energy innovation.
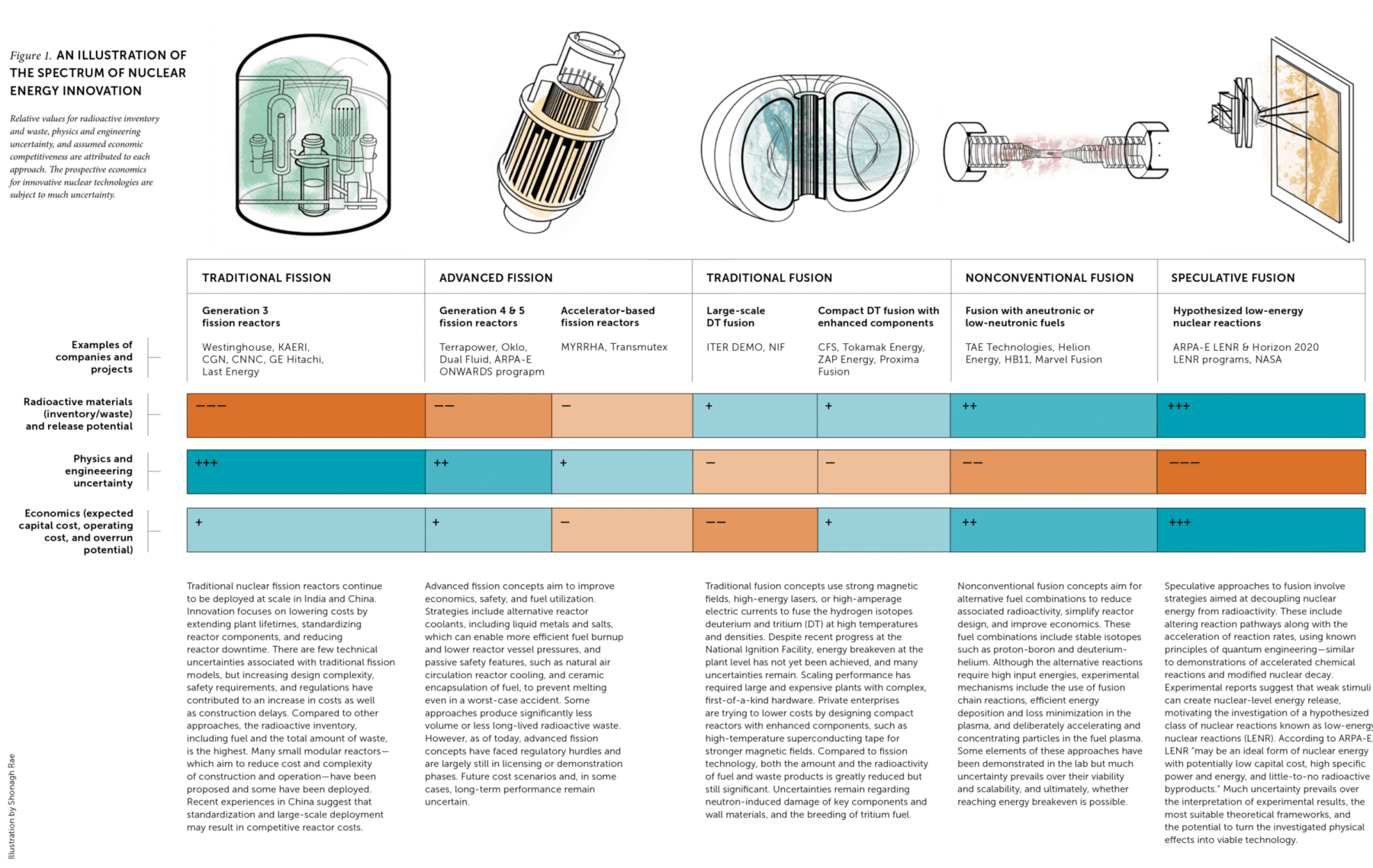
The need for a dynamic portfolio approach
As of yet, there is no consensus about which nuclear technologies will be dominant in the future. Each of the approaches we’ve listed has attracted at least $100 million in funding—and in the case of traditional fusion and fission research, beyond tens of billions and hundreds of billions, respectively. The sheer diversity of approaches—as well as the tenacity with which they continue to be pursued—indicates that this will be a long game. Until greater consensus emerges, a dynamic portfolio strategy that adjusts research and investment priorities periodically will be necessary.
The purpose of a dynamic portfolio is to mitigate and balance out the risks of individual approaches, while benefiting from their potential upsides. But pursuing a portfolio raises the question of weighting: How should research and development resources be allocated across the different technologies?
A fine-grained analysis would treat radioactive inventory, radioactive waste, and release potential separately. However, in practice, the three are largely correlated.
At the local level, the particular politics of nuclear energy will continue to influence the range of preferred innovation activities, leading to different weight preferences in different locales. We anticipate that such distinct concerns will influence regional and national efforts as local players continue to focus their research agendas on particular subsets of the spectrum. These local efforts, forging ahead with specific projects—such as Commonwealth Fusion System’s tokamak demonstrator in the United States or the accelerator-based fission reactor MYRRHA in Belgium—can do a lot to reduce uncertainty and develop better understanding of what works and what does not. In this way, local efforts can avoid paralysis and place bets on competing technologies until there is convergence on a winning approach. And as policymakers, science managers, and other stakeholders decide on how to weigh investments in research to align with national and institutional goals and risk appetites, the whole spectrum of options can be explored around the globe.
However, as these local research agendas with their respective focal points take shape, policymakers must continue to scan the spectrum for emerging alternatives that exhibit the potential to leapfrog existing technologies—and be prepared to jump if warranted. In the management literature, this principle is known as ambidexterity, meaning managers need to simultaneously focus on their existing portfolio while keeping an eye on new developments with disruptive potential. This principle also applies to policymaking: major national fission and fusion programs need to pursue research deeply while scanning the horizon widely. Management scholars recommend that these two complementary focal fields be adopted by different entities to manage the inherent tension between exploiting existing capabilities and exploring new opportunities.
Even if a national portfolio of projects emphasizes one part of the spectrum, other parts should still be watched and evaluated with an open mind, with periodic adjustments to the way resources are allocated. For instance, if one of the proposed mechanisms for scaling the efficiency of proton-boron fusion can be validated, this reduction of scientific uncertainty would boost the attractiveness of the nonconventional fusion part of the portfolio. Alternatively, if traditional fusion experiments discover that high-energy neutron damage is too costly, decisionmakers may wish to invest more in other approaches.
Policymakers must continue to scan the spectrum for emerging alternatives that exhibit the potential to leapfrog existing technologies—and be prepared to jump if warranted.
However, organizations and individuals alike struggle with ambidexterity. Ambidextrous management requires balancing a natural desire to go “all-in” on visualizing the success of a particular approach, with the realization that future technological paradigms and their implications are hard to predict. This balancing is a particular concern for technological approaches on the right side of the spectrum: public research funding in this area has historically tended to be conservative, making it more likely that a program might fail to recognize a breakthrough technology.
In countries that lack expertise in ambidextrous management, national programs risk getting stuck in the all-in mode, without institutional diversity or dynamic reevaluation and readjustment of priorities. For instance, it’s common for European countries to limit their engagement in nuclear fusion research to ITER-related projects and the centralized EUROfusion Consortium. In contrast, US-based institutions dedicated to nuclear energy innovation have achieved some degree of ambidexterity. The Office of Science at the Department of Energy has led funding for research and development in traditional fusion and fission technologies, whereas the innovation agencies ARPA-E and DARPA have shown a greater appetite for funding nonconventional and speculative fusion approaches. These dual strategies have fostered the emergence of a rich ecosystem for nuclear energy innovation, spanning diverse startups, established corporations, universities, and national labs.
Still, there is much room for progress in the US system. We propose formalizing the process of monitoring the spectrum by creating a dedicated organizational body or committee of scientists from a range of academic disciplines. Acting as impartial referees, they could evaluate developments across the emerging technology ecosystem without being champions of any one approach. Such an organization could coordinate information exchange among stakeholders, leading to better appreciation of how progress changes the relative weights of trade-offs across the spectrum. Ideally, if similar national coordinating bodies were established in other countries, they could all could synchronize their findings, gaining insights on local efforts as well as the overall global portfolio. Policymakers and the public should receive regular updates, so they can weigh in on how they value particular advances across the spectrum, enabling further research and investment in promising areas.
Adopting a portfolio-based approach that is guided by impartial coordination bodies is one way to constructively engage with the complex trade-offs, risks, and upsides of emerging approaches to nuclear energy. We advocate greater attention to these aspects of science and innovation policy as they can lead to more effective allocation of resources and faster convergence toward optimal nuclear energy technologies for the future.